\begin{tabular}{l} Done \\ Submit Question \\ Question 4 Certain radioactive material decays in such a way \\ that the mass remaining after \( t \) years is given by the \\ function \\ \( m(t)=175(0.965)^{t} \) \\ where \( m(t) \) is measured in grams. \\ (a) Find the mass at time \( t=0 \). \\ Your answer is \\ (b) How much of the mass remains after 40 years? \\ Your answer is \\ Give your answer to two decimal places. \\ Submit Question \\ \hline\( \checkmark \) Question 5\end{tabular}
Solución de tutoría real
Responder
Solución

¡Inicia sesión para desbloquear respuestas gratis!
Una plataforma de aprendizaje en la que confían millones de estudiantes y profesores reales.

Revisado y aprobado por el equipo de tutoría de UpStudy
The Deep Dive
To find the mass at time \( t=0 \), you simply plug 0 into the function \( m(t) \): 1. \( m(0) = 175(0.965)^0 = 175(1) = 175 \) grams. So, at time \( t = 0 \), the mass is 175 grams. For the mass remaining after 40 years, you substitute \( t = 40 \) into the function: 2. \( m(40) = 175(0.965)^{40} \). Calculating that gives approximately \( m(40) \approx 175 \times 0.1980 \approx 34.65 \) grams when rounded to two decimal places. Therefore, after 40 years, about 34.65 grams of the radioactive material remains. Now, here comes the interesting part! The study of radioactive decay isn’t just limited to fancy equations; it actually helps us understand everything from the age of ancient artifacts through carbon dating to calculating medicine dosages. Isn’t it cool how physics and chemistry can open windows into history? If you ever find yourself dealing with radioactive substances, remember to keep an eye on proper disposal methods! Common mistakes include neglecting half-life calculations, which can lead to overestimating how long a material will remain potent. Always double-check your work to avoid unexpected surprises – unless they’re fun surprises, that is!
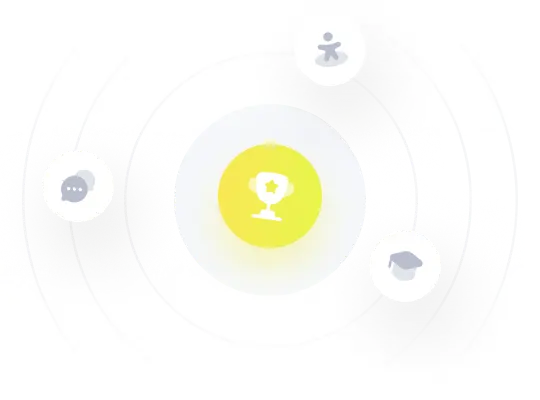